서 론
본 론
희유원소 페그마타이트(rare element pegmatite)와 생산성 화강암(fertile granite)
희유원소 페그마타이트의 분류
LCT형 페그마타이트 탐사 기술
광역 지리정보 취득 및 대상지 위치 선정(GIS regional target selection)
지질조사 및 광물학적 정보 취득(Geological survey and acquisition of primary mineralogical information)
리튬 페그마타이트의 정밀 지화학 분석 및 성인연구(Detailed geochemical analysis and genetic study of lithium pegmatites)
국내 LCT형 페그마타이트 탐사 현황
결 론
서 론
범세계적인 2050 탄소 중립 정책 시행으로 전기 자동차를 비롯한 친환경 산업 시장은 고성장을 지속하고 있으며, 이에 따른 배터리 생산에 대한 수요는 나날이 급증하고 있다. 주요 국가들과 글로벌 기업체들은 배터리 양극재의 핵심 광종인 리튬 수급확보와 관련 산업개발에 주력하고 있으며, 이에 대응하기 위한 리튬 광산에 대한 이해와 탐사 기술에 대한 중요성이 대두되고 있다. 리튬은 기원하는 환경에 따라 페그마타이트에서 추출하는 경암형(hard rock-type), 호수에서 생산하는 염수형(brine-type) 유문암과 같은 화산암에서 유래한 점토광물로부터 추출하는 점토형(clay- type)이 있다(Bradley et al., 2017; Bowell. et al., 2020) (Fig. 1). 최근에는 리튬 추출에 대한 기술력이 향상되면서 점토형 리튬에도 관심이 대두되고 있으나, 해당 광체에 대한 연구가 다른 두 유형에 비하여 미비한 상황인 가운데, 저렴한 생산 원가와 개발 착수 기간에 대한 효율을 고려하여 경암형 방식에 대한 선호가 여전히 큰 추세이다.
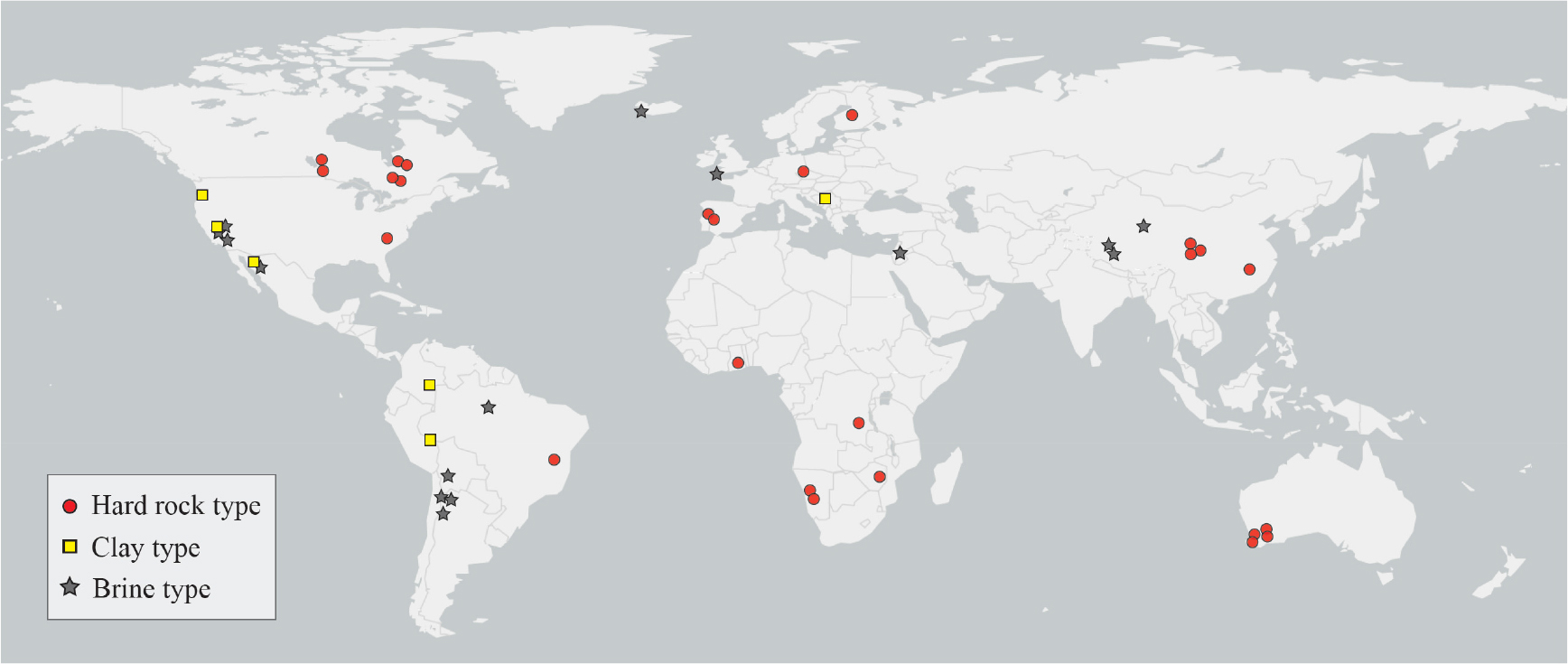
Fig. 1.
World map showing distribution of world-class lithium deposits and geological ore type (modified from Bowell et al., 2020).
리튬을 포함하는 희유원소 페그마타이트를 탐사하기 위해서는 지질광상조사와 더불어 다양한 지구물리탐사 방법이 적용된다. 지질광상조사에서는 희유원소 페그마타이트의 분포 및 규모를 파악하고 자원량을 평가하기 위해 광화작용과 관련된 생산성 화강암(fertile granite)에 대하여 생성 기작, 근원암체(source rock)와의 관계와 이들이 함유한 주원소 및 미량원소의 성분적 특성에 대하여 정밀하게 파악하는 것이 필수적이다. 희유원소 페그마타이트는 생산성 화강암으로부터 거리가 증가함에 따라 지역적 분대(regional zoning)를 나타낸다(Selway et al., 2005)(Fig. 2). 이러한 지역적 분대는 희유원소 페그마타이트의 광물조합, 내부 조직, 지구화학적인 암석의 산출특성 차이로 구분된다. 지표지질조사 또는 시추탐사를 통하여 암석 시료를 획득하고, 이후 채취한 시료를 대상으로 K/Rb, K/Cs, Nb/Ta, Mg/Li 비 확인을 위한 전암분석 및 광물 화학분석(예를 들면, K-장석의 K/Rb, Cs 또는 백운모의 K/Rb, Cs, Li, Ta)을 실시하여 화성암체들의 분화와 결정화작용 발달 양상(fractionation and emplacement patterns) 파악을 통해 희유원소 페그마타이트의 진화 특성을 유추할 수 있다(Selway et al., 2005). 또한, 동위원소 분석(U-Pb, K-Ar, Ar-Ar 등)을 통하여 암석 생성 연대의 측정 및 해석으로 생산성 화강암과 희유원소 페그마타이트의 상관관계를 추정할 수 있다. 함 리튬 페그마타이트 광체에 대한 지구물리탐사는 희유원소 페그마타이트를 대상으로 하여, 광역 탐사(regional exploration; 항공 전자탐사, 항공 초분광탐사, 항공 자력탐사, 항공 방사능탐사)와 지표탐사(전자탐사, GPR탐사, 중력탐사, 전기 비저항탐사)를 수행한다(Table 1). 또한 근래에 탐사기술이 최신화 및 향상되면서 위성영상을 활용하여 희유원소 페그마타이트의 분포 특성을 파악하는 탐사기술 관련 연구들이 보고되고 있다(Son et al., 2015).
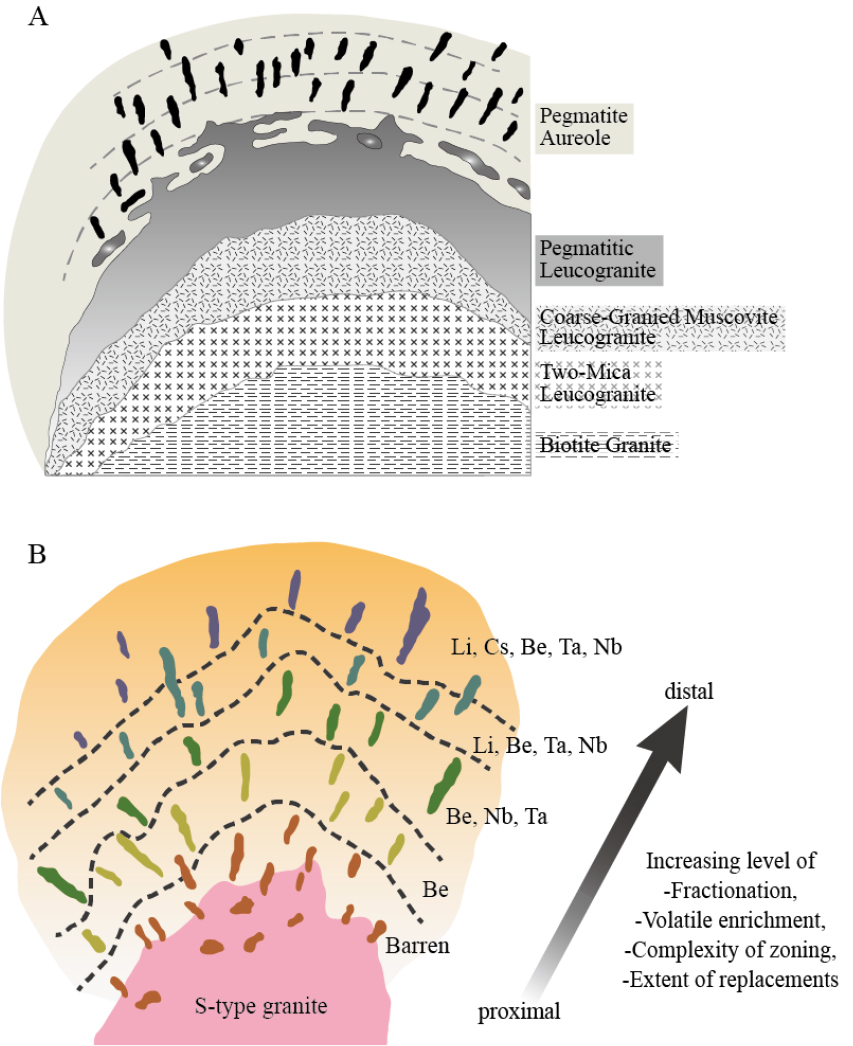
Fig. 2.
Regional zoning in fertile granites and pegmatites (modified from Cerny, 1991b ; Selway et al., 2005). (A) The zonation texture of fertile granite (outward-fractionated) with a halo of external lithium-rich pegmatites; (B) Typical model of regional zoning pattern in syngenetic parent granite + pegmatite group. Rare-element pegmaties demonstrate diverse degrees of fractionation and evolution as they extend from the source granite towards the distal zone.
Table 1.
Exploration techniques to be tested by GREENPEG for identification of LCT and NYF pegmatites (modified from Müller et al., 2022)
희유원소 페그마타이트는 여러 단계를 거듭한 생성학적 과정을 거쳐 형성되므로 페그마타이트 내에 광물의 종류가 다양하여, 리튬(Li) 외에 초전도체, 산화제, 전자기기에 필수적인 세슘(Cs), 탄탈럼(Ta), 니오븀(Nb), 이트륨(Y), 불소(F), 붕소(B), 희토류(REE) 등의 원소들을 포함하고 있어, 해당 광체 탐사에 대한 중요성이 보고된 바 있다. 본 연구에서는 효과적인 희유원소 페그마타이트 광체 탐사를 위하여 관련 탐사 기술을 소개하고, 국내에 분포하는 희유원소 페그마타이트 광상과 잠재자원량 평가를 위한 탐사기술 개발 현황을 소개하고자 한다.
본 론
희유원소 페그마타이트(rare element pegmatite)와 생산성 화강암(fertile granite)
페그마타이트 광상은 마그마의 페그마타이트 시기에 형성되며, 페그마타이트는 일반 페그마타이트와 희유원소 페그마타이트로 구분된다(Selway et al., 2005). 일반 페그마타이트는 주로 석영, 장석, 운모 등 단순한 광물들로 구성되며, 비교적 단기간의 화성활동 또는 변성작용에 의해 형성된다. 희유원소 페그마타이트는 초기 단일 성분의 심성암체로부터 여러 차례 진행된 분별결정화를 통해 다양한 광물을 배태하게 되며, 관련 암상은 다음과 같다(Fig. 2A). 희유원소 페그마타이트는 석영, 장석, 운모뿐만 아니라 Li, Cs, Ta, Nb, Y, F, B, REE 등과 같은 희유원소와 H2O, F–, BO33– 및 PO43– 등의 휘발성분들을 다량 함유하고 있다(Selway et al., 2005).
희유원소 페그마타이트는 생산성 화강암으로부터 대략 10 km 이내에 분포하며(Breaks and Tindle, 1997), 근원암체로부터 분별결정작용의 진행도가 변화하여 다양한 성분적 분대를 이룬다(Selway et al., 2005)(Fig. 2). 생산성 화강암은 대부분 퇴적암 기원(Sedimentary type; S-type granite)의 마그마로부터 생성되며, 마그마 결정화 과정에서 석영, 장석, 운모와 같은 조암광물들과 강한 호정성(compatible)을 나타내는 Si, Al, Na, K 등이 용융체 내에서 고갈되고 상대적으로 후기에는 불호정성(incompatible) 원소들과 휘발성 성분(volatiles)들이 분화도가 높은 용융체 내에 농집되면서 생성된다. 생산성 화강암은 지구화학적, 광물학적 및 조직적인 측면에서 일반적인 화강암과 차이를 나타낸다Selway et al., 2005; Černý and Ercit, 2005). 생산성 화강암은 규소(Si) 함량이 높고, 알루미늄(Al)이 풍부한 광물들(백운모, 석류석, 전기석 등)로 구성되어 지구화학적으로 과알루미나형(peraluminious)의 특징을 보인다(Černý and Ercit, 2005). 연구자들에 의해 제시된 생산성 화강암의 지구화학적 특징은 Table 2와 같다. 이러한 생산성 화강암은 희유원소가 풍부한 특성이 반영되어 다양한 광물들이 산출하며, 흔히 문상구조(graphic texture)를 나타낸다.
Table 2.
Geochemical characteristics of element content and ratios within Li-Sn bearing granitoid (modified from Oh et al., 2022)
Indicators | Criteria | Wangpiri-granite (Uljin area) | References |
SiO2 | ≥72% | 75.4 % | Flinter, 1971; Tischendorf, 1977; Moseley, 1981 |
Sn | >10 ppm | 102 ppm | Flinter, 1971; Biste, 1981; Ivanov and Spomior, 1981 |
Li | >72 ppm | >400 ppm | Biste, 1981; Ivanov and Spomior, 1981 |
Rb | >326 ppm | 748 ppm | Biste, 1981 |
Be | >4 ppm | 10.8 ppm | Ivanov and Spomior, 1981; Moseley, 1981 |
F | >1,415 ppm | 1,700ppm | Biste, 1981; Moseley, 1981 |
Mg/Li | <10 | 2.5 | Selway et al., 2005 |
Nb/Ta | <8 | 2.6 | Selway et al., 2005 |
(Li×1000)/K | >2.4 | 14.6 | Tauson and Kozlov, 1972 |
Ba/Rb | <0.5 | 0.02 | Tauson and Kozlov, 1972 |
K/Rb | <130 | 36.6 | Beus and Grigorian, 1977 |
Rb/Sr | >6 | 91.2 | Govett, 1983 |
(Rb2×Li)/(K×Mg×Sr) | >10-3 | 0.9986 | Govett, 1983 |
Al2O3/(Na2O+K2O+CaO)* | ≥1.1 | 1.5 | Govett, 1983 |
Log(Li×Rb×Be×F×Sn) | >9 | 11.7 | Turekian and Wedepohl, 1961 |
생산성 화강암은 결정화 작용과 함께 마그마의 분화도가 증가하면서 조성에 차이를 나타낸다. 중심부의 초기 흑운모 화강암으로부터 분화가 진행되어 주변부에 이르면서 복운모 우백질 화강암, 거정 백운모 우백질 화강암을 거쳐 가장 외각부에는 거정 우백질 화강암으로 변화한다(Černý and Meintzer, 1988; Černý, 1989)(Fig. 2A). 희유원소 페그마타이트는 분화도가 높은 상부 화강암 주변에 분포하며, 생산성 화강암으로부터 원지성 환경일수록 분화도 증가에 따라 희유원소 및 휘발성분의 함량이 증가하게 되고, 이에 기반하여 지역적 분대를 나타낸다(Černý and Meintzer, 1988; Černý, 1989)(Fig. 2B).
희유원소 페그마타이트의 분류
희유원소 페그마타이트는 우세하게 부화되는 희유원소의 성분적 특성과 지구조 환경의 관계성에 따라 LCT(Li-Cs-Ta; lithium, cesium, tantalum)형 페그마타이트와 NYF (Nb-Y-F; niobium, yttrium and REE, fluorine)형 페그마타이트, 그리고 이들의 성분적 특성을 모두 반영하는 혼합형(Mixed type)으로 분류된다(Černý, 1991a, 1991b; Černý and Ercit, 2005)(Table 3). LCT형 페그마타이트는 Li, Rb, Cs, Be, Sn, Ta>Nb, B, P, F의 원소들이 부화되어 산출하는 것이 특징인 반면, NYF형 페그마타이트는 Nb>Ta, Ti, Y, Sc, REE, Zr, U, Th, F 등이 우세하게 농집된 산상을 보인다(Černý and Ercit, 2005)(Table 3). 각 희유원소 페그마타이트에 부화된 원소의 기원은 다음과 같다. LCT형 페그마타이트는 대륙지각의 섭입대 환경에서 부분용융으로 생성된 변성퇴적암 기원의 S형 화강암을 근원암으로 하며, 고농도의 Si와 Al 함량 특성이 반영되어 흑운모 및 백운모와 인회석의 산출과 함께 각섬석이 결핍된 특성을 보인다(Selway et al., 2005; Černý et al., 2012)(Table 3). 이러한 광물조성은 용융되어 훗날 높은 함수 광물인 K-장석, 알루미노 규산염 및 첨정석(spinel)을 배태한다(Černý et al., 2012). NYF형 페그마타이트의 경우, 비조산대 환경에서 하부 지각 및 맨틀성분으로부터 부분용융으로 생성된 A형 화강암(Anorogenic granites)에서 주로 기원하여, LCT형에 비해 저농도의 Si 및 Al 함량(subaluminous 내지 metaluminous)과 함께 Nb, Y, F, U, Th 및 REE의 원소가 부화되고, LCT형과 달리 Fe 성분이 풍부한 운모류와 각섬석 및 휘석을 동반한 조성 특성을 보인다(Selway et al., 2005)(Table 3). 또한, 상기 언급한 두 유형의 지화학적 특성이 모두 반영된 형태로 혼합형(mixed-type)이 있다(Černý et al., 2012)(Table 3).
Table 3.
The family system of petrogenetic classification of granitic pegmatites of plutonic derivation (modified from Černý, 1991a; Černý and Ercit, 2005).
S형 화강암을 근원암으로 하는 LCT형 페그마타이트는 근원 심성암체로부터 비교적 원지성에서 산출되기 때문에, 분별결정작용 후 원암에서 용리된 유체와 달리, 호정성 원소를 모두 소진한 잔류유체(residual melts)에 부화되는 불호정성 원소성분이 집적된다(Selway et al., 2005; Černý and Ercit, 2005). LCT형 페그마타이트 내 불호정성 원소는 LILE (large-ion lithophile elements; Cs+, Rb+, Ba2+ and Sr2+), HFSE(high field strength elements; Ta5+, Nb5+, P5+ and REE3+), pegmatophile elements(Li+, Be2+ and B3+; Simmons et al., 2003)가 주를 이룬다(Selway et al., 2005; Černý and Ercit, 2005; Steiner, 2019). 이러한 리튬과 밀접한 상관관계를 보이는 원소들은 특정 광물에 집적되어 새로운 함 리튬 광물을 형성하며, 다음에 제시하였다: 스포듀민(spodumene), 엽장석(petalite), 앰블리고석(amblygonite), 홍운모(lepidolite), 진왈다이트(zinnwaldite), 유크립타이트(eucryptite), 주석석(cassiterite), 백운모, 인회석(apatite), 전기석, 탄탈석(tantalite)-컬럼바이트(columbite), 녹주석(beryl), K-장석, 알바이트(albite)(Balaram and Sawant, 2022) (Table 3). 상기 함 리튬 광물은 동일한 생성환경에서 평형을 이루어 함께 산출되는 수반 광물(accessory minerals)과 함께 탐사 지시광물(indicator minerals)로써 적극적으로 활용된다.
NYF형 페그마타이트의 경우, 하부지각과 맨틀로부터 생성된, 석영 내지 장석질 암석의 용융으로 생성된 A형 화강암에서 기원되어, 열점 환경이나 대륙 내 융기대 환경과 밀접한 생성학적 상관관계가 있다. 또한 LCT형에 비하여 함수율이 낮으나 각섬석과 휘석 및 흑운모를 다량 포함한 마그마로부터 F 성분이 부화되어 황옥(topaz)과 형석(fluorite)이 풍부하게 산출한다(Černý et al., 2012).
LCT형 페그마타이트에 대한 광체 탐사는 리튬과 함께 탄탈럼, 주석, 루비듐과 그 외 희유 원소 생산을 위하여, 이들의 지화학적 성분 특성을 고려하여 적절한 탐사기술을 적용해 잠두광체를 탐색한다.
LCT형 페그마타이트 탐사 기술
리튬을 함유하는 페그마타이트는 암석학적-화학적 분류에 따라 LCT(Li-Cs-Ta)형 페그마타이트에 해당하며, LCT형 페그마타이트 탐사를 진행하기 위해서 이행하는 탐사 과정은 다음과 같다(Steiner, 2019)(Fig. 3): 1) 사전 문헌조사(Literature and Desktop study), 2) 광역 지리정보 취득과 대상지 위치 선정(GIS regional target selection), 3) 지질조사 및 광물학적 정보 취득(Geological survey and acquisition of primary mineralogical information), 4) 리튬 페그마타이트의 정밀 지화학 분석 및 성인연구(Detailed geochemical analysis and genetic study of lithium pegmatites).
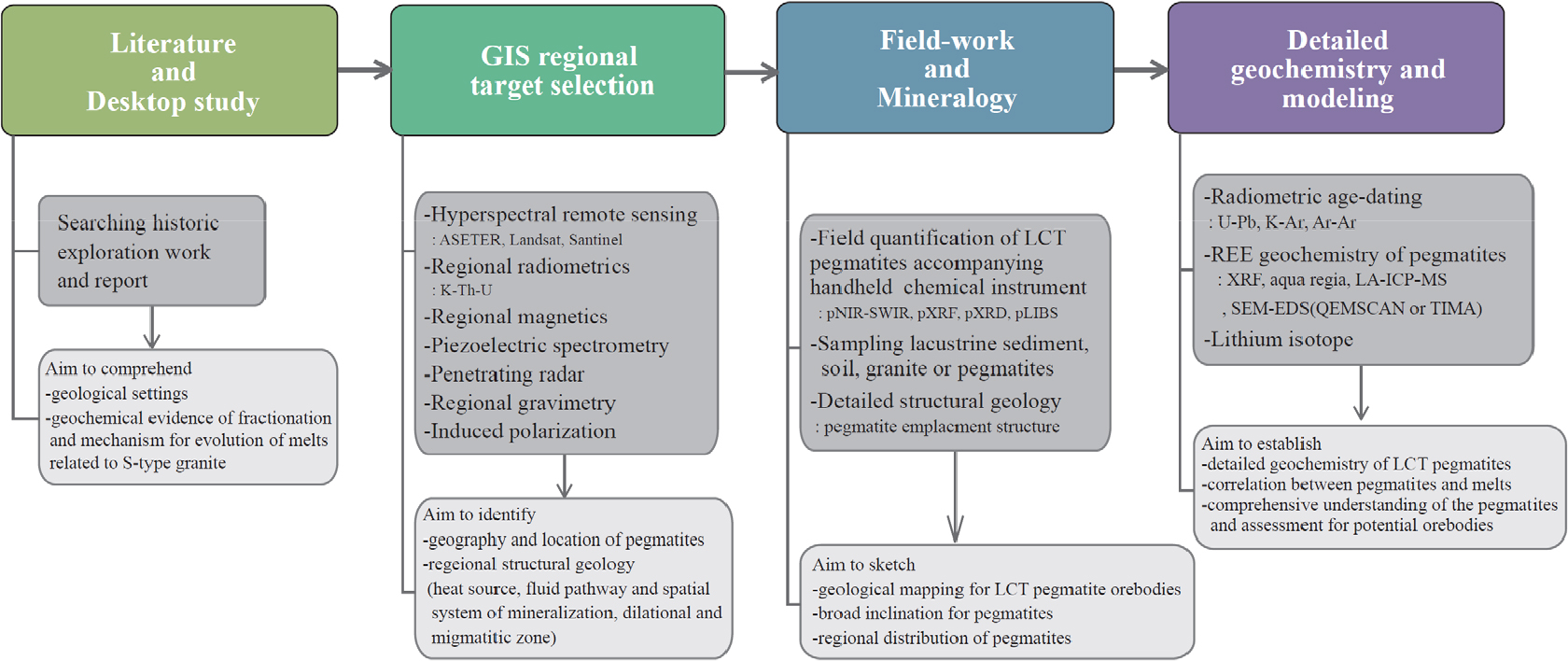
Fig. 3.
Overview of the workflow for the exploration of LCT pegmatites (modified from Steiner, 2019).
사전 문헌조사(Literature and Desktop study)
사전 문헌조사 단계에서는 함 리튬 페그마타이트 광체를 발달시킨 근원암의 형성과 지구조 운동 과정부터 부분용융 및 원소농집을 발달시킨 유체 생성 기작을 아우르는 광체 생성환경에 관한 기존 연구의 전반적인 사전 지식을 습득한다(Steiner, 2019). 문헌조사는 LCT형 페그마타이트 연구지역 일대를 포함한 지역에서 분화한 화강암체 및 페그마타이트를 대상으로 진행된 기존 연구를 조사하며, 산출되는 주요 원소와 미량원소의 거동특성, 마그마 분별결정작용의 산상, 광체 형태(orebody geometry) 및 품위 정보 등을 확인하여 페그마타이트 산출지역에 대한 이해를 도모한다(Steiner, 2019).
광역 지리정보 취득 및 대상지 위치 선정(GIS regional target selection)
문헌 조사 이후 광역 지리정보(GIS; Geographical Information Systems)를 통해 원활한 탐사 수행을 위한 기본적인 지리정보를 탐색하여 광체 대상지에 대한 지리적 정보를 습득하고 지질조사를 위한 준비를 수행하며, 동원되는 물리탐사 기법은 Table 1과 같다. LCT형 페그마타이트 광체는 ASTER, Landsat 및 Sentinel-2 satellite 등의 위성자료를 활용한 열수 변질작용 분포지를 측지 및 가시화(mapping)하여 취득하는 초분광 원격탐사 데이터(Hyperspectral remote sensing data)와 함께 항공 방사능 탐사 및 자력탐사를 수행하여 K-Th-U 분광계측 데이터를 취득하는 과정에서 화강암체에 대한 지리적 위치를 파악하고 광화대와 열원체의 공간적 관계를 이해할 수 있다(Cook et al., 1996; Schetselaar et al., 2000; Steiner, 2019)(Table 1). 자성 및 전도성이 없는 광물로 구성된 리튬 광화대에서는 자성을 통해 직접적으로 광체의 위치를 파악할 수 없으나, 항공자력탐사와 전기 비저항 탐사를 수행하여 화강암질 관입암체 내 발달한 단층대를 비롯한 광역적인 불연속면의 구조를 파악함으로써, 이들 구조대가 제공하는 유체의 이동 통로와 광화작용을 유발한 구조적 특성을 확인할 수 있다. 또한 페그마타이트의 저밀도 특성(≈2.65 g/cm3)을 고려하여 주변 암상과의 밀도 대비를 통해 이상대를 확인할 수 있는 중력탐사도 활용될 수 있다(Müller et al., 2022)(Table 1).
지질조사 및 광물학적 정보 취득(Geological survey and acquisition of primary mineralogical information)
LCT형 페그마타이트 광체에 대한 대상지 지리적 위치에 대한 정보 확인 후 수행되는 단계로, 페그마타이트 암체의 광역적 분포와 마그마 분화작용 및 암체에서 기인된 유체의 생성학적 환경특성을 파악하기 위하여 야외 현장조사를 통해 지질도를 작성하고, 페그마타이트의 생성과 구조지질 및 암상의 화학적 조성 특성을 파악하기 위하여 현장에서 기초 정성 및 정량분석을 진행한다. 이후, 실내 고분해능 분석장비를 갖춘 실험실에서 진행할 정밀 정량분석을 위한 시료를 채취한다(Steiner, 2019).
LCT형 페그마타이트 암체에 대한 직접적인 지질조사를 수행하기에 앞서, 탐사지역 일대를 이루는 수계에 대하여 하상 퇴적물 및 토양시료를 채취하고 기초 지화학 분석을 통해 페그마타이트 내 불호정성 원소와 리튬의 존재를 지시하는 지시원소에 대한 기본적인 정보를 확인한다(Steiner, 2019)(Table 2 and 3). 토양과 하상퇴적물은 이차 분산에 의한 재평형을 이루어 지질학적 정보를 반영하기 때문에 LCT형 페그마타이트 탐사 시 필수적으로 선행되어야 한다. 채취한 토양 및 하상 퇴적물 시료는 압축 펠릿형 XRF(pressed pellets XRF)와 함께 강산 분해를 활용한 화학분석(aqua-regia digests)을 진행하여 리튬을 제외한 이들 시료에 함유된 중광물인 주석석, 엽장석, 컬럼바이트, 탄탈라이트 및 저어콘을 비롯한 규산염 및 산화광물에 대한 용해도 감소와 이온화 반응을 발생시켜 LCT형 페그마타이트의 화학적 조성을 파악한다. 또한, 리튬 원소와 상관관계를 이루는 K/Rb, Nb/Ta 및 Zr/Hf 비를 확인하여 리튬 이상대에 대한 정보를 확인한다(Steiner, 2019).
LCT형 페그마타이트 광체에 대한 정밀 야외 지질조사는 지리정보와 하상 퇴적물 기초 지화학 탐사에서 확인한 Li, Cs, Ta, Nb, Sn, W, Be, B 원소 이상대를 대상으로 현장 지질조사를 실시한다. 하지만 현장 노두에서는 함 리튬 광물의 산출 특성이 조암광물과 유사하여, 육안으로 명확히 구별하기 어렵다. 따라서 Table 4에 예시된 휴대용 화학 분석기기를 동원하여 함 리튬 광물과 함께 산출하는 지시원소의 화학성분을 파악함으로써, LCT형 페그마타이트에 대한 전반적인 품위와 근원암에 대한 전반적인 조성 특성을 파악한다. 이러한 휴대용 분석기기는 탐사 목표로 주력하는 특정 원소의 이온 전자가 크기와 전하량에 따라 다양하게 활용될 수 있다. 야외지질조사 현장에서 흔하게 활용되는 pXRF의 경우, LCT형 페그마타이트의 주요 지시원소인 Be과 Li와 같은 전하량(Z) 13 이하의 경원소(lighter elements)에 대한 정성분석은 불가한 점을 고려해, pLIBS를 동반하여 Be, Li 분석과 함께 Li의 존재를 암시하는 지시원소인 Sn, W, Ta, K, Rb를 함께 확인함으로써, 원소들의 상관관계를 파악하여 분석 한계를 보완한다(Balaram and Sawant, 2022)(Table 4).
Table 4.
Summary of optimum portable geochemical tools for in-situ LCT pegmatite exploration (modified from Balaram and Sawant, 2022)
Name of Chemical Handheld Instrument | Main feature |
Pathfinder mineral |
Detectable element focusing on lithium-bearing exploration |
pNIR-SWIR |
-determining the mineral species, composition and crystallinity of clay, carbonate and sulfate minerals by measuring the wavelengths of infrared light absorbed present in rock samples |
lepidolite, spodumene | - REEs, Nd |
pXRF |
-measuring characteristic radiations at a particular wavelength or energy to determine elements (both qualitative and quantitative) within the sample -undetectable to measure Li and Be (due to low level of X-ray energy levels to reach the detector) |
cassiterite, columbite-tantalite |
: Cs, Ta, Nb, Sn, W, K, Rb ; detectable Z>13 |
pXRD |
-identifying and quantifying a wider range of minerals compared to either SWIR or Raman spectroscopy through the crystallinity |
lithium-bearing silicates, cassiterite, sulfides |
ore grade Nb-Ta oxides, Li-bearing silicate minerals |
pLIBS |
-detecting a short and high-energy laser pulses from rock samples, creating a plasma of small amounts of ablated materials -detectable for lighter elements such as Be and Li, which is not possible through pXRF |
lithium-bearing silicates | -Mg, Na, C, B, Be, Li |
리튬 페그마타이트의 정밀 지화학 분석 및 성인연구(Detailed geochemical analysis and genetic study of lithium pegmatites)
휴대용 화학분석을 통해 확인한 함 리튬 LCT형 페그마타이트 부광대에서 채취한 암석 시료는 관입 심성암체의 화학적 성분이 리튬을 배태하기 위한 과알루미나형 및 생산성 화강암인지 확인하기 위하여 화강암 및 반화강암을 대상으로 전암분석을 수행하는데, 이 단계에서 이들 암석 내 산출광물 내 희유원소의 성분 조성(Mg/Li, Nb/Tb 비, Li, Cs, Rb)을 파악한다(Selway et al., 2005). LCT형 페그마타이트의 위치와 분대 확인 이후, 광물학적 조성을 정밀하게 확인하기 위하여 정성 및 정량적 화학분석, 그리고 페그마타이트 내 산출하는 주요 함 리튬 광물을 대상으로 동위원소 연령측정(U-Pb, K-Ar, 40Ar/39Ar)을 실시한다(Bradley et al., 2017)(Fig. 3). 전암 분석 및 정성 및 정량 화학분석에 주로 사용되는 분석기법은 XRD, SEM-EDS(QEMSCAN; Quantitative Evaluation of Minerals by Scanning Electron Microscopy 혹은 TIMA; TESCAN Integrated Mineral Analyzer)를 활용하고, 광물 개별 단위에 대한 주원소 분석 및 미량원소 정밀분석과 조성비 데이터를 획득하고자, EPMA(electron probe microanalyzer), LA-ICP-MS(laser-ablation inductively coupled plasma-mass spectrometry) 분석이 활용된다(Steiner, 2019; Wells et al., 2022). LCT형 페그마타이트는 근원암체로부터 다양한 분대구조를 광역적으로 이루는 특성으로 인해 일반적인 전암분석용 암석 시료를 통해 XRF 혹은 LA-ICP-MS 분석을 진행할 경우, 광체 정보를 충분히 반영하지 못하고 균일하지 않은 화학 조성으로 분석될 수 있어, 분대구조 발달방향에 수직으로 시추한 코어를 대상으로 정밀 분석을 진행한다(Steiner, 2019; Müller et al., 2022). LA-ICP-MS 미량원소데이터는 페그마타이트에 수반되는 저어콘에서 U-Pb와 함께 함 리튬 광물 및 운모류를 대상으로 분석한 K-Ar, Ar-Ar 원소 비 확인으로 지질연대 확인을 통해 리튬 형성과 밀접한 연관성을 보이는 페그마타이트의 분포와 생성시기를 추정하여, 함 리튬 페그마타이트와 근원 마그마의 상관성을 파악하는 중요한 탐사지침이 될 수 있다. 또한 주요 지시광물 내 K/Rb, Zr/Hf, Nb/Ta 비와 Li원소의 동위원소(6Li/7Li) 비를 활용하여 초기원암으로부터 발달한 분대구조에 대해, 광체의 생성기작과 기원을 규명할 수 있다(Steiner, 2019; Wells et al., 2022).
국내 LCT형 페그마타이트 탐사 현황
국내 LCT형 페그마타이트는 경북 울진, 충북 제천, 충북 단양, 강원 춘천에 분포하는 것으로 보고된 바 있다(Shibata, 1969; Moon et al., 1996; Oh et al., 2022). 가행된 광산은 경북 울진의 보암광산에서 1945년에서 1963년까지 약 18년간 180 Mt를 채굴한 기록이 유일하며, 보암광산의 리튬(LiO2) 품위는 1.69-1.90 % 로 보고되었다(Korea Institute of Geology, Mining & Materials, 1991). 울진 일대 함 리튬 페그마타이트는 선캠브리아기 화강편마암, 변성퇴적암 또는 퇴적암을 관입하였다. 함 리튬 페그마타이트는 맥상(dyke) 또는 관입암상(sill)으로 분포하며, 폭이 수십 m 이내로 좁고 연장은 최대 수백 m 정도로 추정되고 있다. Lee et al.(2013)은 울진 일대 보암광산에서 관찰되는 함 리튬 페그마타이트를 동광체, 본광체, 서광체로 구분하였으며, Oh et al.(2022)은 이들 광체가 광물조합의 차이에 따라 지역적 분대를 나타낸다고 제시하였다. 또한, 함 리튬 페그마타이트는 서광체보다 동광체에 가까울수록 내부 분대(internal zoning)를 보이며, 리튬 광화작용은 페그마타이트 내부에만 국한된다. 보암광산 주변 함 리튬 페그마타이트의 형태와 분포는 단층, 습곡, 층 경계와 같은 지질구조에 규제되며, ENE-WSW 방향으로 우세하게 분포하고 있다(KIGAM, 2021). 보암광산에서 북서쪽으로 약 1 km 떨어진 곳에서는 압쇄화 작용(mylonitization)을 받은 함 리튬 페그마타이트가 분포한다(KIGAM, 2021)(Fig. 4). 이 함 리튬 페그마타이트는 선캠브리아기의 변성퇴적암(율리층군)을 관입한 우백질 화강편마암(분천화강편마암 또는 왕피리화강편마암) 주변에 분포하며 화강편마암과 같은 NE 방향을 보인다. Moon and Park(1994)은 상기 우백질 화강편마암의 높은 희유원소 함량(Li, 229-1,092 ppm; Rb, 510-910 ppm; F, 2,000-7,600 ppm)을 근거로 분천화강편마암(1,863 ± 103 Ma; Lee, 1988)과 상이한 지구화학 특성을 보고하였다.
현장 탐사에서는 앞서 소개한 LCT형 페그마타이트 탐사 기술을 참고하여 문헌조사 결과와 더불어 광역 지구화학탐사와 지구물리탐사(항공 방사능탐사, 중력탐사)를 수행하고 탐사적지(왕피리화강편마암과 보암광산 일대)를 선정하였다(Fig. 5). 이후 탐사적지 내 함 리튬 페그마타이트 생성과 관련한 생산성 화강암(즉, 관계화성암) 기원 파악을 위하여 Breaks and Tindle(1997)이 제시한 페그마타이트 생성연구를 기반으로 탐사대상지(314 km2)를 중심으로 지구화학적 벡터링 연구가 수행되었다(KIGAM, 2021)(Table 2). 일반적으로 함 리튬 페그마타이트와 관련된 생산성 화강암은 Table 2와 같은 지구화학적 조성 특성을 보이는데, 연구지역의 우백질 화강편마암(왕피리화강편마암)은 Table 2에 제시한 15개의 기준을 모두 충족하고 있다. 중력탐사 결과에서는 우백질 화강편마암과 보암광산 주변에 상대적으로 낮은 중력이상을 나타내고 있어(Fig. 5), 지표에 드러나지 않은 화성암체의 분포가 예상되며(KIGAM, 2021), 현재 3차원 역산과 모델링을 통해 이 잠두 화성암체의 분포와 규모를 파악 중이다. 지표지질조사에서는 함 리튬 페그마타이트의 2차원 분포를 파악하였으며(Fig. 4), 광물조합, 광체조직, 전암분석 결과를 바탕으로 페그마타이트의 지역적 분대를 구분하여 생산성 화강암의 방향(보암광산 서광체 방향)을 추정하였다. 또한, 광물 지화학분석 결과를 이용하여 울진 일대 페그마타이트의 분화패턴을 연구하고 생산성 화강암의 분포를 추정할 계획이다.
결 론
희유원소 페그마타이트는 Li, Cs, Ta, Nb, Y, F, B, REE 등과 같은 희유원소와 휘발성 물질이 풍부하고 여러 단계에 걸쳐 형성되어 다양한 핵심광물을 포함한다. 하지만, 대부분의 희유원소 페그마타이트는 오랜 기간 변형 또는 풍화되어 평형을 이룬 변성암 지대에 발달하여 지표 관찰 및 추적 과정에서의 한계성과 맥상 또는 관입암상으로 발달하는 소규모 광체로 타 광상에 비하여 부광대 파악이 제한적이다. 따라서 효과적인 희유원소 페그마타이트 탐사를 위해서는 LCT 페그마타이트의 산출 특성과 함께 리튬의 광상학적 지식을 바탕으로 다양한 지질조사 및 물리탐사 자료를 통합한 체계적인 해석 기법이 동원되어야 한다. LCT형 페그마타이트 대상 탐사 단계는 크게 사전 문헌조사, 광역지리정보 취득과 대상지 위치 선정, 지질조사 및 기초 광물학적 정보취득, 리튬 페그마타이트의 정밀 지화학 분석 및 성인연구의 네 가지로 구성된다. 국내 부존한 함 리튬 페그마타이트 탐사 사례를 종합한 주요 탐사 기술들은 다음과 같다.
(1) 생산성 화강암 확인: 연구지역에서 희유원소 페그마타이트와 생산성 화강암의 분포를 파악하고, 광물 관찰 및 전암분석을 통하여 마그마의 기원(S-type)과 지구화학적 특성을 확인할 필요가 있다. 만약, 지표에서 생산성 화강암 확인이 어려운 경우에는 지구화학탐사 또는 시추탐사를 통해 분포 특성 및 규모를 추정할 필요가 있다.
(2) 희유원소 페그마타이트의 지역적 분대 확인: 희유원소 페그마타이트는 생산성 화강암으로부터 대략 10 km 이내에 분포하며, 생산성 화강암으로부터 거리가 증가함에 따라 지역적 분대를 나타낸다.
(3) 분화도 확인: 전암분석 원소 파악(K/Rb, K/Cs, Nb/Ta, Mg/Li) 및 광물 화학분석(K-장석의 K/Rb, Cs 또는 백운모의 K/Rb, Cs, Li, Ta)을 실시하여 화성암체들의 분화패턴을 파악하면 희유원소 페그마타이트의 발달 방향을 추정할 수 있다.
(4) 연대 측정: 생산성 화강암과 희유원소 페그마타이트의 생성 연대 확인하여야 한다.
(5) 지구물리탐사: 희유원소 페그마타이트 탐사를 위해 항공 전자탐사, 항공 초분광탐사, 항공 자력탐사, 항공 방사능탐사와 같은 광역탐사와 전자탐사, GPR탐사, 중력탐사, 전기 비저항탐사와 같은 지표탐사를 상황에 맞게 수행하여 지질광상자료와 함께 복합적으로 해석할 필요가 있다.