Introduction
Hazard Identification and Risk Assessment
Hazard identification
Risk Assessment
Methodology for Risk Management: Bowtie Method
Bowtie Method
A Model for Mountain Tunnel Risk Management: Addressing Geotechnical Hazards using the Bowtie Method
Conclusions
Introduction
In South Korea, tunnel constructions have progressively become longer and deeper. While many of these projects have been executed safely, there have been instances of delays, cost overruns, and, unfortunately, more severe consequences such as injuries and loss of life. To mitigate these risks and enhance safety, it is imperative to systematically assess and manage the hazards inherent in tunnel construction.
Here are some examples of risk assessment in tunnel construction from academic papers:
• Multiscale Evaluation of Tunnel Construction Safety Risk: A Case Study of an Offshore Tunnel Construction in Ningbo: This study identifies the risk factors associated with the construction of an offshore tunnel foundation pit in Ningbo using the WBS-RBS method. The fuzzy comprehensive evaluation method is then applied to assess the project’s construction safety risk (Wu et al., 2024).
• Safety Risk Assessment of Loess Tunnel Construction Under Complex Environment Based on Game Theory-Cloud Model: This paper analyzes the safety risk factors in the construction of loess tunnels, specifically focusing on the Luochuan tunnel project of the Xi’an–Yan’an High-Speed Railway. It uses a combination of game theory and cloud model to establish a risk assessment system (Han et al., 2023).
• Risk Analysis During Tunnel Construction Using Bayesian Networks: Porto Metro Case Study: This research utilizes Bayesian networks to analyze the risks associated with the construction of the Porto Metro tunnel (Rita and Herbert, 2012).
These papers provide various methodologies and case studies on risk assessment in tunnel construction projects.
In risk assessment, the terms ‘hazards’ and ‘risks’ are often used interchangeably, making it essential to clearly distinguish between them (Sahu and Paithannkar, 2011)
• A hazard is anything that has the potential to cause harm.
• The risk is how likely it is that a hazard will cause actual harm.
Before risks can be dealt with, they must be identified, characterized and quantified. As human judgment is not only based on evidence, but also on experience and anecdotal knowledge, lay assessments of risks appear to be heavily influenced by individual risk perception. Thus, ‘risk’ can both relate to an objective reality and to a subjective way of interpretation. Understanding and influencing the individual perception may help to prevent the manifestation of the risk under consideration (Michalsen, 2010).
In the field of tunnel construction, risk management can be broadly divided into two main areas: risk analysis and risk control. Risk analysis involves identifying, assessing, and understanding the potential hazards and risks associated with tunnel construction projects. This includes evaluating the likelihood and impact of various risk events, such as geological instabilities, equipment failures, and environmental factors.
On the other hand, risk control focuses on implementing measures to mitigate or eliminate the identified risks. This involves developing and applying strategies, procedures, and technologies to prevent risk events from occurring or to minimize their impact if they do occur. Examples of risk control measures include reinforcing tunnel linings, conducting regular safety inspections, and implementing emergency response plans.
However, as observed in the reviewed literature, most studies on tunnel construction risks tend to emphasize risk analysis, often neglecting the equally important aspect of risk control. This imbalance highlights a gap in the current research and practice, where the development and application of effective risk control techniques are not given sufficient attention.
Therefore, this paper aims to address this gap by adopting a methodological approach to risk control in tunnel construction. It will explore various risk control strategies, evaluate their effectiveness, and provide practical recommendations for their implementation. By doing so, the paper seeks to contribute to a more comprehensive understanding of risk management in tunnel construction, ensuring that both risk analysis and risk control are adequately addressed.
In Chapter 3, the paper discusses a risk management methodology based on the bowtie method. The bowtie method is commonly used in safety and risk assessment to visualize and analyze potential hazards, their causes, and the barriers in place to prevent or mitigate them. It’s a powerful tool for understanding risk scenarios.
The chapter likely covers how to construct a bowtie diagram, identify critical control points, and assess the effectiveness of preventive and mitigative measures.
Chapter 4 introduces a risk management model specifically tailored for mountainous tunnels. Given the unique challenges posed by tunnel construction and operation in mountainous terrain, this model likely addresses factors such as geological instability.
The use of the bowtie method in this context demonstrates its practicality and applicability to real-world scenarios.
In the concluding chapter (Chapter 5), the paper likely summarizes the findings and implications of the risk management approach discussed in the preceding chapters.
It may also highlight any recommendations for practitioners, further research directions, or practical insights gained from applying the bowtie method to mountainous tunnel risk management.
Hazard Identification and Risk Assessment
Hazard identification
The purpose of hazard identification is to identify and develop a list of hazards for each job in the organization that are reasonably likely to expose people to injury, illness or disease if not effectively controlled. Workers can then be informed of these hazards and controls put in place to protect workers prior to them being exposed to the actual hazard. If one does not properly identify the problem then it becomes difficult to assess the risk or postulate solutions. Hazard identification takes persistence to characterize known hazards and creativity to identify the new ways the system design or operation can lead to an accident (Hardy, 2010).
There are several approaches typically used to help identify hazards (Ericson, 2005). One approach that tends not to be used extensively is the review of accident reports from different industries and applications. Analyses of accident reports help identify flaws in existing hazard analyses processes and help discover hazards that might not have been considered. Hazard identification should be undertaken systematically, aiming to cover the full field of possibilities. Most techniques for identifying hazards meet this principle by subdividing the operation or equipment into appropriate number of elements, and for each element to be considered in turn against a comprehensive checklist of possible problems, mishaps, abnormalities, etc. It is good practice to include in the team people with a variety of technical expertise, and from varying levels in the organization (Department of Minerals Resources, 1997).
Geotechnical event during tunnel construction
This study systematically collected historical and potential risk data for identification purposes. The data were sourced from both domestic and international disaster-related statistics, scholarly papers, reliability reports, and comprehensive literature reviews.
In 2010, a database of 204 tunnel construction accidents was assembled by Rita. This is the most comprehensive database known to date. The database was analyzed to better understand the causes of accidents. Based on the data collected the main observed accidents are presented in Table 1.
Table 1.
Adverse tunnel-construction events (Rita, 2010)
Fig. 1 illustrates the distribution of undesirable events within the database. Notably, the majority of reported events pertain to collapses and daylight collapses (36% and 28%, respectively). However, it is essential to clarify that these reported events do not necessarily represent the actual prevalence of incidents during tunneling construction. Rather, they are frequently documented in the literature and emphasized by experts due to their potentially severe consequences for the construction process, worker safety, and the well-being of individuals and surface structures
Fig. 2 presents the statistical distribution of tunnel events based on the Case Histories of tunnel collapses in Korea (Korean Tunnel Association, 2010). Notably, the order of incidence for tunnel events during construction is as follows: rock falls, daylight collapses, collapses, flooding, excessive deformation, and other related occurrences.
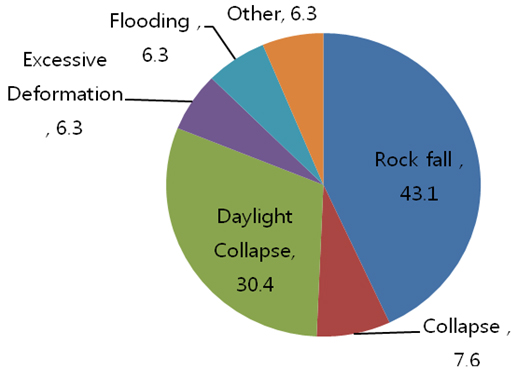
Fig. 2.
Distribution of adverse tunnel-event distributions in Korea (Korean Tunnel Association, 2010).
Risk Assessment
The objective of risk analysis is to produce outputs that can be used to evaluate the nature and distribution of risk and to develop appropriate strategies to manage risk. Events or issues with more significant consequences and likelihood are identified as ‘higher risk’ and are selected for higher priority mitigation actions to lower the likelihood of the event happening and reduce the consequences if the event were to occur.
Qualitative approaches to risk assessment are the most commonly applied (O’Beirne and Napper, 1990). This is because there is a lack of accurate, valid ‘hard’ data about event likelihood; there is a wealth of industry experience at the management, supervisory and operational levels that can suggest subjective consequence and event likelihood; most of the time the objective of the risk assessment is to manage priority risks, an objective that does not require a quantitative approach for an effective outcome.
A logical systematic process is usually followed during a qualitative risk assessment to identify the key risk events and to assess the consequences of the events occurring and the likelihood of their occurrence.
Consequence
The consequence can be considered as the “worst case scenario” or outcome that can reasonably be expected should an incident occur. Consequences can generally occur in two areas: personal injury and property/process damage or loss. To assist in determining consequences the criteria adopted in this study was presented in Table 2.
Table 2.
Consequences of adverse tunnel-construction events
Likelihood
The likelihood, often called probability, of an incident occurring is largely dependent on the frequency of exposure. The following aspects should be considered when making this decision:
• The number of times tasks/cycles/situations occur
• The number of people performing the tasks or exposed, and
• What has happened in the past here or elsewhere in similar situations.
The following criteria were adopted as a guideline for risk likelihood (Table 3).
Table 3.
Likelihood criteria of tunnel-construction risks
Risk matrix
Once the consequences 0 to 5 and likelihood A to E are selected, a single risk rating can be selected from the risk classification matrix as presented in Fig. 3.
Methodology for Risk Management: Bowtie Method
The BowTie methodology is used for risk assessment, risk management and (very important) risk communication. The method is designed to give a better overview of the situation in which certain risks are present; to help people understand the relationship between the risks and organizational events.
The strength of the methodology lies in its simplicity; the phrase “less is more” is certainly applicable.
Risk management is all about risk-perception management, since most accidents happen because of actions or inactions of people. People working in hazardous environments should be aware of the present organizational risks and should have an accurate understanding of their role in it. This can only be accomplished by sufficient risk communication adjusted to the abilities of that part of the workforce you want to address, leading to the establishment of operational ownership.
Many risk assessments are done using quantitative instruments. These may be sufficient for certain types of equipment but are less valuable for organizational risk assessment. Human beings are less easy to predict than machinery and the operational combination of all factors present (think of people, equipment, time, weather, organizational factors, etc.) leads to even more difficulties.
Bowtie Method
Risk in BowTie methodology is elaborated by the relationship between Hazards, Top Events, Threats and Consequences. Controls are used to display what measures an organization has in place to control the risk (CGE Risk, 2012).
Fig. 4 illustrates the concept of the bowtie method. At the center, the hazard and top event define the risks we need to control. On the left side, threats and the barriers to prevent them are depicted, while on the right side, consequences and the controls to mitigate them are shown
Hazard
The word “Hazard” suggests that it is unwanted, but in fact it is the opposite: it is exactly the thing you want or even need to make business. It is an entity with the potential to cause harm but without it there is no business. For example the oil industry; oil is a dangerous substance (and can cause a lot of harm when treated without care) but it is the one the thing that keeps the oil industry in business! It needs to be managed because as long as it is under control, it is of no harm (CGE Risk, 2012).
Top Event
Thus as long as a hazard is controlled it is in its wanted state. For example: oil in a pipe on its way to shore. But certain events can cause the hazard to be released. In BowTie methodology such an event is called the Top Event. The top event is not a catastrophe yet, but the dangerous characteristics of the hazard are now in the open. For example: oil is outside of the pipeline (loss of containment). Not a major disaster, but if not mitigated correctly it can result in more unwanted events (consequences) (CGE Risk, 2012).
Threats
Often there are several factors that could cause the Top Event. In BowTie methodology these are called Threats. These threats need to be sufficient or necessary: every threat itself should have the ability to cause the Top Event. For example: corrosion of the pipeline can lead to the loss of containment (CGE Risk, 2012).
Consequences
When a Top Event has occurred it can lead to certain consequences. A consequence is a potential event resulting from the release of the Hazard which results directly in loss or damage. Consequences in BowTie methodology are unwanted events that an organization ‘by all means’ wants to avoid. For example: oil leaking into the environment (Risk, 2012).
Barriers / Controls
Risk management involves controlling risks by implementing barriers to prevent certain events from occurring. A Control can be any measure taken that acts against some undesirable force or intention, in order to maintain a desired state. In the BowTie methodology, proactive controls (located on the left side of the Top Event or Barrier) are implemented to prevent the Top Event from occurring. For example, regular corrosion inspections of pipelines. There are also reactive Controls (on the right side of the Top Event) that prevent the Top Event resulting into unwanted consequences. For example: leak detection equipment or concrete floor around oil tank platform (CGE Risk, 2012).
A Model for Mountain Tunnel Risk Management: Addressing Geotechnical Hazards using the Bowtie Method
Based on an analysis of 204 tunnel incidents worldwide and 46 cases of tunnel collapse specifically in Korea, the following geotechnical hazards can be identified:
• Portal Area (face/slope):
- Hazards: Inflow or falling of materials, rock fall, collapse, etc.
- Description: This area is particularly vulnerable to material inflow or falling due to the exposed nature of the tunnel entrance. Rock falls and collapses are common due to the instability of the face and slope.
• Main Section (face in discontinuity and/or weak zone):
- Hazards: Inflow or falling of materials, cave-in/rock fall, inflow of soil from the face, excessive deformation/cracks on shotcrete lining, etc.
- Description: In zones of discontinuity or weakness, the tunnel face is prone to material inflow and falling. Cave-ins and rock falls are frequent, and the shotcrete lining may exhibit excessive deformation or cracks due to the unstable ground conditions.
• Main Section (face/roof in shallow depth and valley area):
- Hazards: Inflow or falling of materials, flow-out of groundwater from the face, sudden failure of the face, sliding in the sidewall caused by joints and water, etc.
- Description: In shallow depth and valley areas, the face and roof of the tunnel are susceptible to material inflow and falling. Groundwater may flow out from the face, leading to sudden failures. The presence of joints and water can cause sliding in the sidewalls.
• Main Section (roof after face advance):
- Hazards: Inflow or falling of materials, sinkhole type failure, etc.
- Description: After the face advances, the roof of the tunnel can experience material inflow and falling. Sinkhole-type failures are also a significant risk in this section.
For each of the four geotechnical hazards mentioned above, we have defined the specific events, established treatments and consequences, and derived barriers and controls to prevent them. The risk management model using the bowtie method is illustrated in Fig. 5, 6, 7, 8 and detailed in Table 4. Table 4 provides a detailed summary of the components of the bowtie method illustrated in Fig. 5, 6, 7, 8, including threats, barriers, controls, and consequences.
Table 4.
Risk-management model for mountain tunnels
This model provides a comprehensive approach to identifying, assessing, and controlling risks in tunnel construction, ensuring that appropriate measures are in place to mitigate potential hazards.
Conclusions
This study analyzed 204 tunnel incidents worldwide and 46 cases of tunnel collapse specifically in Korea to identify major geotechnical hazards in tunnel construction. Through this analysis, we defined specific hazards in the portal area, main section in discontinuity and/or weak zones, main section in shallow depth and valley areas, and the main section after face advance. For each hazard, we established specific events, treatments, and consequences.
Using the bowtie method, we constructed a risk management model, identifying barriers and controls for each hazard. This model provides a comprehensive approach to identifying, assessing, and controlling risks in tunnel construction, ensuring that appropriate mitigation measures are in place.
Therefore, this paper presents a methodological approach to risk control in tunnel construction, offering practical solutions to effectively manage and prevent geotechnical hazards. By doing so, it aims to enhance the safety of tunnel construction projects and promote a balanced approach to risk management.